The asthenosphere from Ancient Greek ἀσθενός asthenós without strength is the mechanically weak and ductile region of th
Asthenosphere
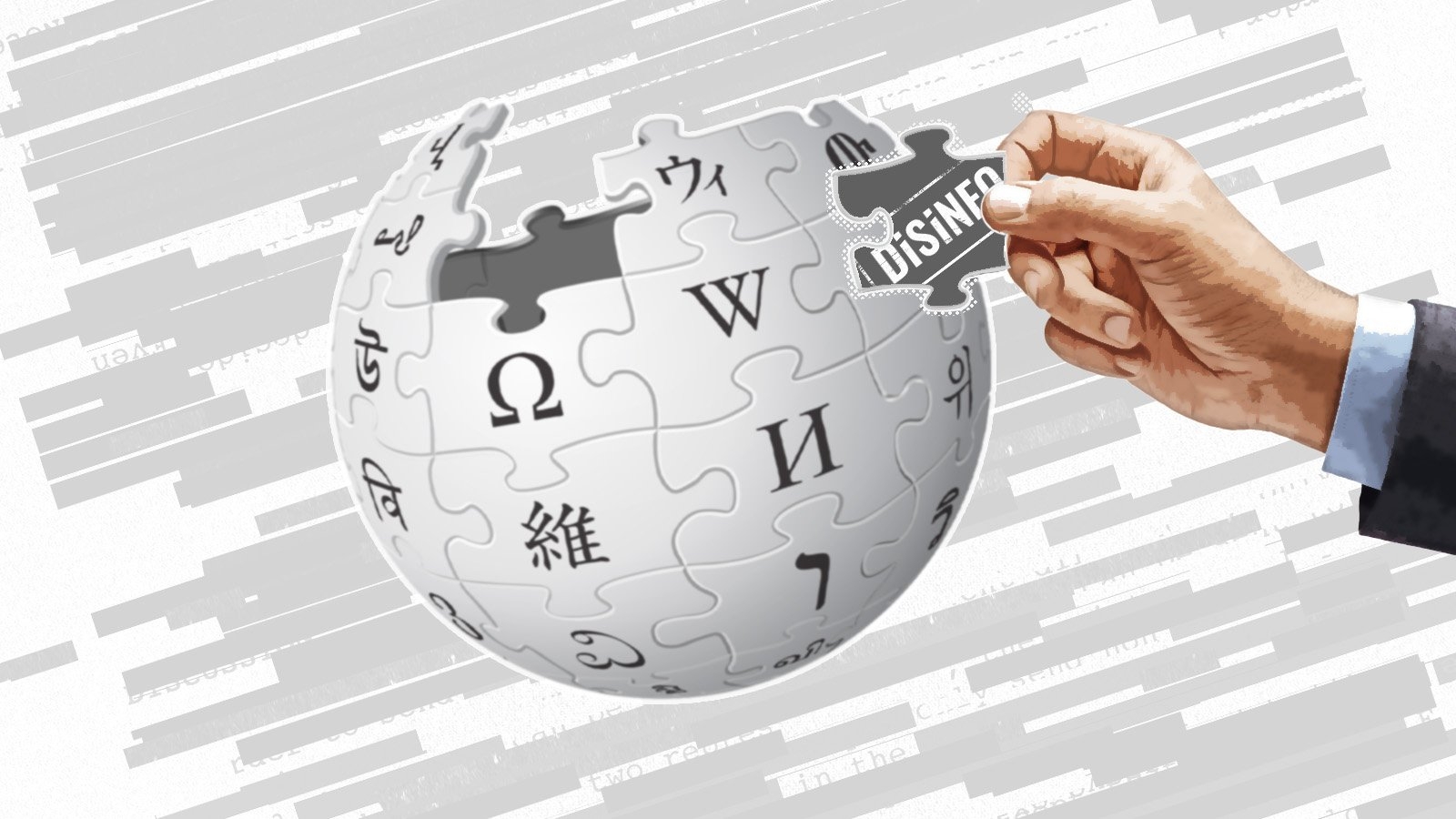
The asthenosphere (from Ancient Greek ἀσθενός (asthenós) 'without strength') is the mechanically weak and ductile region of the upper mantle of Earth. It lies below the lithosphere, at a depth between c. 80 and 200 km (50 and 120 mi) below the surface, and extends as deep as 700 km (430 mi). However, the lower boundary of the asthenosphere is not well defined.

The asthenosphere is almost solid, but a slight amount of melting (less than 0.1% of the rock) contributes to its mechanical weakness. More extensive decompression melting of the asthenosphere takes place where it wells upwards, and this is the most important source of magma on Earth. It is the source of mid-ocean ridge basalt (MORB) and of some magmas that erupt above subduction zones or in regions of continental rifting.
Characteristics

The asthenosphere is a part of the upper mantle just below the lithosphere that is involved in plate tectonic movement and isostatic adjustments. It is composed of peridotite, a rock containing mostly the minerals olivine and pyroxene. The lithosphere-asthenosphere boundary is conventionally taken at the 1,300 °C (2,370 °F) isotherm. Closer to the surface at lower temperatures, the mantle behaves rigidly; deeper below the surface at higher temperatures, the mantle moves in a ductile fashion. The asthenosphere is where the mantle rock most closely approaches its melting point, and a small amount of melt is likely to present in this layer.
Seismic waves pass relatively slowly through the asthenosphere compared to the overlying lithospheric mantle. Thus, it has been called the low-velocity zone (LVZ), although the two are not strictly the same; the lower boundary of the LVZ lies at a depth of 180 to 220 kilometers (110 to 140 mi), whereas the base of the asthenosphere lies at a depth of about 700 kilometers (430 mi). The LVZ also has a high seismic attenuation (seismic waves moving through the asthenosphere lose energy) and significant anisotropy (shear waves polarized vertically have a lower velocity than shear waves polarized horizontally). The discovery of the LVZ alerted seismologists to the existence of the asthenosphere and gave some information about its physical properties, as the speed of seismic waves decreases with decreasing rigidity. This decrease in seismic wave velocity from the lithosphere to the asthenosphere could be caused by the presence of a very small percentage of melt in the asthenosphere, though since the asthenosphere transmits S waves, it cannot be fully melted.
In the oceanic mantle, the transition from the lithosphere to the asthenosphere (the LAB) is shallower than for the continental mantle (about 60 km in some old oceanic regions) with a sharp and large velocity drop (5–10%). At the mid-ocean ridges, the LAB rises to within a few kilometers of the ocean floor.
The upper part of the asthenosphere is believed to be the zone upon which the great rigid and brittle lithospheric plates of the Earth's crust move about. Due to the temperature and pressure conditions in the asthenosphere, rock becomes ductile, moving at rates of deformation measured in cm/yr over lineal distances eventually measuring thousands of kilometers. In this way, it flows like a convection current, radiating heat outward from the Earth's interior. Above the asthenosphere, at the same rate of deformation, rock behaves elastically and, being brittle, can break, causing faults. The rigid lithosphere is thought to "float" or move about on the slowly flowing asthenosphere, enabling isostatic equilibrium and allowing the movement of tectonic plates.
Boundaries
The asthenosphere extends from an upper boundary at approximately 80 to 200 km (50 to 120 miles) below the surface to a lower boundary at a depth of approximately 700 kilometers (430 mi).
Lithosphere-asthenosphere boundary
The lithosphere-asthenosphere boundary (LAB) is relatively sharp and likely coincides with the onset of partial melting or a change in composition or anisotropy. Various definitions of the boundary reflect various aspects of the boundary region. In addition to the mechanical boundary defined by seismic data, which reflects the transition from the rigid lithosphere to ductile asthenosphere, these include a thermal boundary layer, above which heat is transported by thermal conduction and below which heat transfer is mainly convective; a rheological boundary, where the viscosity drops below about 1021 Pa-s; and a chemical boundary layer, above which the mantle rock is depleted in volatiles and enriched in magnesium relative to the rock below.
Lower boundary of asthenosphere
The lower boundary of the asthenosphere, the top of the tentatively defined mesosphere or mesospheric shell, is less well-defined, but has been placed at the base of the upper mantle. This boundary is neither seismically sharp nor well understood but is approximately coincident with the complex 670 km discontinuity. This discontinuity is generally linked to the transition from mantle rock containing ringwoodite to mantle rock containing bridgmanite and periclase.
Origin
The mechanical properties of the asthenosphere are widely attributed to the partial melting of the rock. It is likely that a small amount of melt is present through much of the asthenosphere, where it is stabilized by the traces of volatiles (water and carbon dioxide) present in the mantle rock. However, the likely amount of melt, not more than about 0.1% of the rock, seems inadequate to fully explain the existence of the asthenosphere. This is not enough melt to fully wet grain boundaries in the rock, and the effects of melt on the mechanical properties of the rock are not expected to be significant if the grain boundaries are not fully wetted. The sharp lithosphere-asthenosphere boundary is also difficult to explain by partial melting alone. It is possible that the asthenosphere is a zone of minimum water solubility in mantle minerals so that more water is available to form greater quantities of melt. Another possible mechanism for producing mechanical weakness is grain boundary sliding, where grains slide slightly past each other under stress, lubricated by the traces of volatiles present. Weakening below oceanic plates is partly caused by their motion itself, thanks to the non-linear dislocation creep mechanism.
Numerical models of mantle convection in which the viscosity is dependent both on temperature and strain rate reliably produce an oceanic asthenosphere, suggesting that strain-rate weakening is a significant contributing mechanism, and explaining the particularly weak asthenosphere below the Pacific plate.
Magma generation
Decompression melting of asthenospheric rock creeping towards the surface is the most important source of magma on Earth. Most of this erupts at mid-ocean ridges to form the distinctive mid-ocean ridge basalt (MORB) of the ocean crust. Magmas are also generated by decompressional melting of the asthenosphere above subduction zones and in areas of continental rifting.
Decompression melting in upwelling asthenosphere likely begins at a depth as great as 100 to 150 kilometers (60 to 90 mi), where the small amounts of volatiles in the mantle rock (about 100 ppm of water and 60 ppm of carbon dioxide) assist in melting not more than about 0.1% of the rock. At a depth of about 70 kilometers (40 mi), dry melting conditions are reached and melting increases substantially. This dehydrates the remaining solid rock and is likely the origin of the chemically depleted lithosphere.
See also
- Seismology § History
References
- Barrell, J. (1914). "The strength of the crust, Part VI. Relations of isostatic movements to a sphere of weakness – the asthenosphere". The Journal of Geology. 22 (7): 655–83. Bibcode:1914JG.....22..655B. doi:10.1086/622181. JSTOR 30060774. S2CID 224832862.
- Hirschmann 2010.
- Self, Steve; Rampino, Mike (2012). "The crust and lithosphere". Geological Society of London. Retrieved 27 January 2013.
- Kearey, Klepeis & Vine 2009, p. 49.
- Forsyth, Donald W. (1975). "The early structural evolution and anisotropy of the oceanic upper mantle". Geophysical Journal International. 43 (1): 103–162. Bibcode:1975GeoJ...43..103F. doi:10.1111/j.1365-246X.1975.tb00630.x.
- Kearey, P., ed. (1993). The Encyclopedia of the Solid Earth Sciences. Oxford: Blackwell Science. ISBN 978-1-4443-1388-8. OCLC 655917296.
- Eppelbaum, Lev V.; Kutasov, I.M.; Pilchin, Arkady (2013). Applied Geothermics. Berlin, Germany. ISBN 978-3-642-34023-9. OCLC 879327163.
{{cite book}}
: CS1 maint: location missing publisher (link) - Condie, Kent C. (1997). Plate Tectonics and Crustal Evolution. Butterworth-Heinemann. p. 123. ISBN 978-0-7506-3386-4. Retrieved 21 May 2010.
- Kearey, Klepeis & Vine 2009, p. 51.
- Karato 2012.
- Rychert, Catherine A.; Shearer, Peter M. (2011). "Imaging the lithosphere-asthenosphere boundary beneath the Pacific using SS waveform modeling". Journal of Geophysical Research: Solid Earth. 116 (B7): B07307. Bibcode:2011JGRB..116.7307R. doi:10.1029/2010JB008070.
- Kearey, Klepeis & Vine 2009, pp. 48–49.
- Hendrix, Mark; Thompson, Graham R.; Turk, Jonathan (2015). Earth (2nd ed.). Stamford, CT. ISBN 978-1-285-44226-6. OCLC 864788835.
{{cite book}}
: CS1 maint: location missing publisher (link) - Garrison, Tom; Ellis, Robert (2017). Essentials of Oceanography (8th ed.). Pacific Grove, CA. ISBN 978-1-337-51538-2. OCLC 1100670264.
{{cite book}}
: CS1 maint: location missing publisher (link) - Gupta, Harsh K., ed. (2011). Encyclopedia of Solid Earth Geophysics. Dordrecht, Netherlands: Springer. ISBN 978-90-481-8702-7. OCLC 745002805.
- Rychert, Catherine A.; Shearer, Peter M. (24 April 2009). "A global view of the lithosphere-asthenosphere boundary". Science. 324 (5926): 495–498. Bibcode:2009Sci...324..495R. doi:10.1126/science.1169754. PMID 19390041. S2CID 329976.
- Artemieva, Irina (2011). The Lithosphere. pp. 6, 12. doi:10.1017/CBO9780511975417. ISBN 978-0-511-97541-7.
- Daly, Reginald Aldworth (1940). Strength and Structure of the Earth. Prentice-Hall.
- Anderson, Don L. (1995). "Lithosphere, asthenosphere, and perisphere". Reviews of Geophysics. 33 (1): 125–149. Bibcode:1995RvGeo..33..125A. doi:10.1029/94RG02785. ISSN 8755-1209.
- Fowler, C.M.R.; Fowler, Connie May (2005). The Solid Earth: An introduction to global geophysics. Cambridge University Press. ISBN 978-0521893077.
- Ito, E; Takahashi, E (1989). "Postspinel transformations in the system Mg2SiO4-Fe2SiO4 and some geophysical implications". Journal of Geophysical Research: Solid Earth. 94 (B8): 10637–10646. Bibcode:1989JGR....9410637I. doi:10.1029/jb094ib08p10637.
- Mierdel, Katrin; Keppler, Hans; Smyth, Joseph R.; Langenhorst, Falko (19 January 2007). "Water solubility in aluminous orthopyroxene and the origin of Earth's asthenosphere". Science. 315 (5810): 364–368. Bibcode:2007Sci...315..364M. doi:10.1126/science.1135422. PMID 17234945. S2CID 33006157.
- Patočka, Čížková & Pokorný 2024.
- Becker, Thorsten W. (November 2006). "On the effect of temperature and strain-rate dependent viscosity on global mantle flow, net rotation, and plate-driving forces". Geophysical Journal International. 167 (2): 943–957. Bibcode:2006GeoJI.167..943B. doi:10.1111/j.1365-246X.2006.03172.x.
- Connolly, James A.D.; Schmidt, Max W.; Solferino, Giulio; Bagdassarov, Nikolai (November 2009). "Permeability of asthenospheric mantle and melt extraction rates at mid-ocean ridges". Nature. 462 (7270): 209–212. Bibcode:2009Natur.462..209C. doi:10.1038/nature08517. PMID 19907492. S2CID 4352616.
- Olive, Jean-Arthur; Dublanchet, Pierre (November 2020). "Controls on the magmatic fraction of extension at mid-ocean ridges". Earth and Planetary Science Letters. 549: 116541. Bibcode:2020E&PSL.54916541O. doi:10.1016/j.epsl.2020.116541. S2CID 224923541.
- Hofmann, A. W. (1997). "Mantle geochemistry: The message from oceanic volcanism". Nature. 385 (6613): 219–228. Bibcode:1997Natur.385..219H. doi:10.1038/385219a0. S2CID 11405514.
- Conder, James A.; Wiens, Douglas A.; Morris, Julie (August 2002). "On the decompression melting structure at volcanic arcs and back-arc spreading centers: Arc and back-arc melting". Geophysical Research Letters. 29 (15): 17–1–17-4. doi:10.1029/2002GL015390. S2CID 29842432.
- Keen, C.E.; Courtney, R.C.; Dehler, S.A.; Williamson, M.-C. (February 1994). "Decompression melting at rifted margins: comparison of model predictions with the distribution of igneous rocks on the eastern Canadian margin". Earth and Planetary Science Letters. 121 (3–4): 403–416. Bibcode:1994E&PSL.121..403K. doi:10.1016/0012-821X(94)90080-9.
- Sternai, Pietro (December 2020). "Surface processes forcing on extensional rock melting". Scientific Reports. 10 (1): 7711. Bibcode:2020NatSR..10.7711S. doi:10.1038/s41598-020-63920-w. PMC 7206043. PMID 32382159.
Bibliography
- Hirschmann, Marc M. (March 2010). "Partial melt in the oceanic low velocity zone". Physics of the Earth and Planetary Interiors. 179 (1–2): 60–71. Bibcode:2010PEPI..179...60H. doi:10.1016/j.pepi.2009.12.003.
- Karato, Shun-ichiro (March 2012). "On the origin of the asthenosphere". Earth and Planetary Science Letters. 321–322: 95–103. Bibcode:2012E&PSL.321...95K. doi:10.1016/j.epsl.2012.01.001.
- Patočka, Vojtěch; Čížková, Hana; Pokorný, J. (12 November 2024). "Dynamic Component of the Asthenosphere: Lateral Viscosity Variations Due to Dislocation Creep at the Base of Oceanic Plates". Geophysical Research Letters. 51 (13). Bibcode:2024GeoRL..5109116P. doi:10.1029/2024GL109116.
- Kearey, P.; Klepeis, Keith A.; Vine, F.J. (2009). Global Tectonics (3rd ed.). Oxford: Wiley-Blackwell. ISBN 978-1-4051-0777-8. OCLC 132681514.
- McBride, Neil; Gilmour, Iain (2004). An Introduction to the Solar System. Cambridge University Press. ISBN 978-0-521-54620-1. Retrieved 24 January 2016.
- Turcotte, Donald L.; Schubert, Gerald (2002). Geodynamics (2nd ed.). Cambridge University Press. ISBN 978-0-521-66624-4. Retrieved 24 January 2016.
External links
- "The Earth's internal heat energy and interior structure". Geology. Department of Earth & Environmental Sciences. San Diego, CA: San Diego State University. Archived from the original on 3 March 2011. Retrieved 20 July 2024.
Author: www.NiNa.Az
Publication date:
wikipedia, wiki, book, books, library, article, read, download, free, free download, mp3, video, mp4, 3gp, jpg, jpeg, gif, png, picture, music, song, movie, book, game, games, mobile, phone, android, ios, apple, mobile phone, samsung, iphone, xiomi, xiaomi, redmi, honor, oppo, nokia, sonya, mi, pc, web, computer
The asthenosphere from Ancient Greek ἀs8enos asthenos without strength is the mechanically weak and ductile region of the upper mantle of Earth It lies below the lithosphere at a depth between c 80 and 200 km 50 and 120 mi below the surface and extends as deep as 700 km 430 mi However the lower boundary of the asthenosphere is not well defined The asthenosphere shown at a subduction boundary The asthenosphere is almost solid but a slight amount of melting less than 0 1 of the rock contributes to its mechanical weakness More extensive decompression melting of the asthenosphere takes place where it wells upwards and this is the most important source of magma on Earth It is the source of mid ocean ridge basalt MORB and of some magmas that erupt above subduction zones or in regions of continental rifting CharacteristicsThe asthenosphere in relation to the other layers of Earth s structure The asthenosphere is a part of the upper mantle just below the lithosphere that is involved in plate tectonic movement and isostatic adjustments It is composed of peridotite a rock containing mostly the minerals olivine and pyroxene The lithosphere asthenosphere boundary is conventionally taken at the 1 300 C 2 370 F isotherm Closer to the surface at lower temperatures the mantle behaves rigidly deeper below the surface at higher temperatures the mantle moves in a ductile fashion The asthenosphere is where the mantle rock most closely approaches its melting point and a small amount of melt is likely to present in this layer Seismic waves pass relatively slowly through the asthenosphere compared to the overlying lithospheric mantle Thus it has been called the low velocity zone LVZ although the two are not strictly the same the lower boundary of the LVZ lies at a depth of 180 to 220 kilometers 110 to 140 mi whereas the base of the asthenosphere lies at a depth of about 700 kilometers 430 mi The LVZ also has a high seismic attenuation seismic waves moving through the asthenosphere lose energy and significant anisotropy shear waves polarized vertically have a lower velocity than shear waves polarized horizontally The discovery of the LVZ alerted seismologists to the existence of the asthenosphere and gave some information about its physical properties as the speed of seismic waves decreases with decreasing rigidity This decrease in seismic wave velocity from the lithosphere to the asthenosphere could be caused by the presence of a very small percentage of melt in the asthenosphere though since the asthenosphere transmits S waves it cannot be fully melted In the oceanic mantle the transition from the lithosphere to the asthenosphere the LAB is shallower than for the continental mantle about 60 km in some old oceanic regions with a sharp and large velocity drop 5 10 At the mid ocean ridges the LAB rises to within a few kilometers of the ocean floor The upper part of the asthenosphere is believed to be the zone upon which the great rigid and brittle lithospheric plates of the Earth s crust move about Due to the temperature and pressure conditions in the asthenosphere rock becomes ductile moving at rates of deformation measured in cm yr over lineal distances eventually measuring thousands of kilometers In this way it flows like a convection current radiating heat outward from the Earth s interior Above the asthenosphere at the same rate of deformation rock behaves elastically and being brittle can break causing faults The rigid lithosphere is thought to float or move about on the slowly flowing asthenosphere enabling isostatic equilibrium and allowing the movement of tectonic plates BoundariesThe asthenosphere extends from an upper boundary at approximately 80 to 200 km 50 to 120 miles below the surface to a lower boundary at a depth of approximately 700 kilometers 430 mi Lithosphere asthenosphere boundary The lithosphere asthenosphere boundary LAB is relatively sharp and likely coincides with the onset of partial melting or a change in composition or anisotropy Various definitions of the boundary reflect various aspects of the boundary region In addition to the mechanical boundary defined by seismic data which reflects the transition from the rigid lithosphere to ductile asthenosphere these include a thermal boundary layer above which heat is transported by thermal conduction and below which heat transfer is mainly convective a rheological boundary where the viscosity drops below about 1021 Pa s and a chemical boundary layer above which the mantle rock is depleted in volatiles and enriched in magnesium relative to the rock below Lower boundary of asthenosphere The lower boundary of the asthenosphere the top of the tentatively defined mesosphere or mesospheric shell is less well defined but has been placed at the base of the upper mantle This boundary is neither seismically sharp nor well understood but is approximately coincident with the complex 670 km discontinuity This discontinuity is generally linked to the transition from mantle rock containing ringwoodite to mantle rock containing bridgmanite and periclase OriginThe mechanical properties of the asthenosphere are widely attributed to the partial melting of the rock It is likely that a small amount of melt is present through much of the asthenosphere where it is stabilized by the traces of volatiles water and carbon dioxide present in the mantle rock However the likely amount of melt not more than about 0 1 of the rock seems inadequate to fully explain the existence of the asthenosphere This is not enough melt to fully wet grain boundaries in the rock and the effects of melt on the mechanical properties of the rock are not expected to be significant if the grain boundaries are not fully wetted The sharp lithosphere asthenosphere boundary is also difficult to explain by partial melting alone It is possible that the asthenosphere is a zone of minimum water solubility in mantle minerals so that more water is available to form greater quantities of melt Another possible mechanism for producing mechanical weakness is grain boundary sliding where grains slide slightly past each other under stress lubricated by the traces of volatiles present Weakening below oceanic plates is partly caused by their motion itself thanks to the non linear dislocation creep mechanism Numerical models of mantle convection in which the viscosity is dependent both on temperature and strain rate reliably produce an oceanic asthenosphere suggesting that strain rate weakening is a significant contributing mechanism and explaining the particularly weak asthenosphere below the Pacific plate Magma generationDecompression melting of asthenospheric rock creeping towards the surface is the most important source of magma on Earth Most of this erupts at mid ocean ridges to form the distinctive mid ocean ridge basalt MORB of the ocean crust Magmas are also generated by decompressional melting of the asthenosphere above subduction zones and in areas of continental rifting Decompression melting in upwelling asthenosphere likely begins at a depth as great as 100 to 150 kilometers 60 to 90 mi where the small amounts of volatiles in the mantle rock about 100 ppm of water and 60 ppm of carbon dioxide assist in melting not more than about 0 1 of the rock At a depth of about 70 kilometers 40 mi dry melting conditions are reached and melting increases substantially This dehydrates the remaining solid rock and is likely the origin of the chemically depleted lithosphere See alsoSeismology HistoryReferencesBarrell J 1914 The strength of the crust Part VI Relations of isostatic movements to a sphere of weakness the asthenosphere The Journal of Geology 22 7 655 83 Bibcode 1914JG 22 655B doi 10 1086 622181 JSTOR 30060774 S2CID 224832862 Hirschmann 2010 Self Steve Rampino Mike 2012 The crust and lithosphere Geological Society of London Retrieved 27 January 2013 Kearey Klepeis amp Vine 2009 p 49 Forsyth Donald W 1975 The early structural evolution and anisotropy of the oceanic upper mantle Geophysical Journal International 43 1 103 162 Bibcode 1975GeoJ 43 103F doi 10 1111 j 1365 246X 1975 tb00630 x Kearey P ed 1993 The Encyclopedia of the Solid Earth Sciences Oxford Blackwell Science ISBN 978 1 4443 1388 8 OCLC 655917296 Eppelbaum Lev V Kutasov I M Pilchin Arkady 2013 Applied Geothermics Berlin Germany ISBN 978 3 642 34023 9 OCLC 879327163 a href wiki Template Cite book title Template Cite book cite book a CS1 maint location missing publisher link Condie Kent C 1997 Plate Tectonics and Crustal Evolution Butterworth Heinemann p 123 ISBN 978 0 7506 3386 4 Retrieved 21 May 2010 Kearey Klepeis amp Vine 2009 p 51 Karato 2012 Rychert Catherine A Shearer Peter M 2011 Imaging the lithosphere asthenosphere boundary beneath the Pacific using SS waveform modeling Journal of Geophysical Research Solid Earth 116 B7 B07307 Bibcode 2011JGRB 116 7307R doi 10 1029 2010JB008070 Kearey Klepeis amp Vine 2009 pp 48 49 Hendrix Mark Thompson Graham R Turk Jonathan 2015 Earth 2nd ed Stamford CT ISBN 978 1 285 44226 6 OCLC 864788835 a href wiki Template Cite book title Template Cite book cite book a CS1 maint location missing publisher link Garrison Tom Ellis Robert 2017 Essentials of Oceanography 8th ed Pacific Grove CA ISBN 978 1 337 51538 2 OCLC 1100670264 a href wiki Template Cite book title Template Cite book cite book a CS1 maint location missing publisher link Gupta Harsh K ed 2011 Encyclopedia of Solid Earth Geophysics Dordrecht Netherlands Springer ISBN 978 90 481 8702 7 OCLC 745002805 Rychert Catherine A Shearer Peter M 24 April 2009 A global view of the lithosphere asthenosphere boundary Science 324 5926 495 498 Bibcode 2009Sci 324 495R doi 10 1126 science 1169754 PMID 19390041 S2CID 329976 Artemieva Irina 2011 The Lithosphere pp 6 12 doi 10 1017 CBO9780511975417 ISBN 978 0 511 97541 7 Daly Reginald Aldworth 1940 Strength and Structure of the Earth Prentice Hall Anderson Don L 1995 Lithosphere asthenosphere and perisphere Reviews of Geophysics 33 1 125 149 Bibcode 1995RvGeo 33 125A doi 10 1029 94RG02785 ISSN 8755 1209 Fowler C M R Fowler Connie May 2005 The Solid Earth An introduction to global geophysics Cambridge University Press ISBN 978 0521893077 Ito E Takahashi E 1989 Postspinel transformations in the system Mg2SiO4 Fe2SiO4 and some geophysical implications Journal of Geophysical Research Solid Earth 94 B8 10637 10646 Bibcode 1989JGR 9410637I doi 10 1029 jb094ib08p10637 Mierdel Katrin Keppler Hans Smyth Joseph R Langenhorst Falko 19 January 2007 Water solubility in aluminous orthopyroxene and the origin of Earth s asthenosphere Science 315 5810 364 368 Bibcode 2007Sci 315 364M doi 10 1126 science 1135422 PMID 17234945 S2CID 33006157 Patocka Cizkova amp Pokorny 2024 Becker Thorsten W November 2006 On the effect of temperature and strain rate dependent viscosity on global mantle flow net rotation and plate driving forces Geophysical Journal International 167 2 943 957 Bibcode 2006GeoJI 167 943B doi 10 1111 j 1365 246X 2006 03172 x Connolly James A D Schmidt Max W Solferino Giulio Bagdassarov Nikolai November 2009 Permeability of asthenospheric mantle and melt extraction rates at mid ocean ridges Nature 462 7270 209 212 Bibcode 2009Natur 462 209C doi 10 1038 nature08517 PMID 19907492 S2CID 4352616 Olive Jean Arthur Dublanchet Pierre November 2020 Controls on the magmatic fraction of extension at mid ocean ridges Earth and Planetary Science Letters 549 116541 Bibcode 2020E amp PSL 54916541O doi 10 1016 j epsl 2020 116541 S2CID 224923541 Hofmann A W 1997 Mantle geochemistry The message from oceanic volcanism Nature 385 6613 219 228 Bibcode 1997Natur 385 219H doi 10 1038 385219a0 S2CID 11405514 Conder James A Wiens Douglas A Morris Julie August 2002 On the decompression melting structure at volcanic arcs and back arc spreading centers Arc and back arc melting Geophysical Research Letters 29 15 17 1 17 4 doi 10 1029 2002GL015390 S2CID 29842432 Keen C E Courtney R C Dehler S A Williamson M C February 1994 Decompression melting at rifted margins comparison of model predictions with the distribution of igneous rocks on the eastern Canadian margin Earth and Planetary Science Letters 121 3 4 403 416 Bibcode 1994E amp PSL 121 403K doi 10 1016 0012 821X 94 90080 9 Sternai Pietro December 2020 Surface processes forcing on extensional rock melting Scientific Reports 10 1 7711 Bibcode 2020NatSR 10 7711S doi 10 1038 s41598 020 63920 w PMC 7206043 PMID 32382159 BibliographyHirschmann Marc M March 2010 Partial melt in the oceanic low velocity zone Physics of the Earth and Planetary Interiors 179 1 2 60 71 Bibcode 2010PEPI 179 60H doi 10 1016 j pepi 2009 12 003 Karato Shun ichiro March 2012 On the origin of the asthenosphere Earth and Planetary Science Letters 321 322 95 103 Bibcode 2012E amp PSL 321 95K doi 10 1016 j epsl 2012 01 001 Patocka Vojtech Cizkova Hana Pokorny J 12 November 2024 Dynamic Component of the Asthenosphere Lateral Viscosity Variations Due to Dislocation Creep at the Base of Oceanic Plates Geophysical Research Letters 51 13 Bibcode 2024GeoRL 5109116P doi 10 1029 2024GL109116 Kearey P Klepeis Keith A Vine F J 2009 Global Tectonics 3rd ed Oxford Wiley Blackwell ISBN 978 1 4051 0777 8 OCLC 132681514 McBride Neil Gilmour Iain 2004 An Introduction to the Solar System Cambridge University Press ISBN 978 0 521 54620 1 Retrieved 24 January 2016 Turcotte Donald L Schubert Gerald 2002 Geodynamics 2nd ed Cambridge University Press ISBN 978 0 521 66624 4 Retrieved 24 January 2016 External links The Earth s internal heat energy and interior structure Geology Department of Earth amp Environmental Sciences San Diego CA San Diego State University Archived from the original on 3 March 2011 Retrieved 20 July 2024